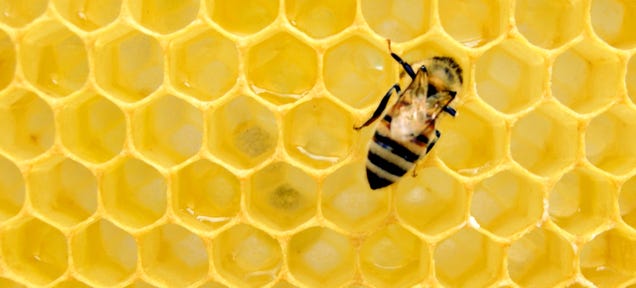
Ellie Lobel was ready to die. Then she was attacked by bees. Christie Wilcox hears how venom can be a saviour.
"I moved to California to die."
Ellie Lobel was 27 when she was bitten by a tick and contracted Lyme
disease. And she was not yet 45 when she decided to give up fighting for
survival.
Caused by corkscrew-shaped bacteria called Borrelia burgdorferi,
which enter the body through the bite of a tick, Lyme disease is
diagnosed in around 300,000 people every year in the United States. It
kills almost none of these people, and is by and large curable – if
caught in time. If doctors correctly identify the cause of the illness
early on, antibiotics can wipe out the bacteria quickly before they
spread through the heart, joints and nervous system.
But back in the spring of 1996, Ellie didn't know to look for the
characteristic bull's-eye rash when she was bitten – she thought it was
just a weird spider bite. Then came three months with flu-like symptoms
and horrible pains that moved around the body. Ellie was a fit, active
woman with three kids, but her body did not know how to handle this
new invader. She was incapacitated. "It was all I could do to get my
head up off the pillow," Ellie remembers.
Her first doctor told her it was just a virus, and it would run its
course. So did the next. As time wore on, Ellie went to doctor after
doctor, each giving her a different diagnosis. Multiple sclerosis.
Lupus. Rheumatoid arthritis. Fibromyalgia. None of them realised she was
infected with Borrelia until more than a year after she
contracted the disease – and by then, it was far too late. Lyme bacteria
are exceptionally good at adapting, with some evidence that they may
be capable of dodging both the immune system and the arsenal of
antibiotics currently available. Borrelia are able to live all
over the body, including the brain, leading to neurological symptoms.
And even with antibiotic treatment, 10–20 per cent of patients don't get
better right away. There are testimonies of symptoms persisting –
sometimes even resurfacing decades after the initial infection – though
the exact cause of such post-treatment Lyme disease syndrome is a topic
of debate among Lyme scientists.
"I just kept doing this treatment and that treatment," says Ellie. Her
condition was constantly worsening. She describes being stuck in bed
or a wheelchair, not being able to think clearly, feeling like she'd
lost her short-term memory and not feeling "smart" anymore. Ellie kept
fighting, with every antibiotic, every pharmaceutical, every holistic
treatment she could find. "With some things I would get better for a
little while, and then I would just relapse right back into this
horrible Lyme nightmare. And with every relapse it got worse."
After fifteen years, she gave up.
"Nothing was working any more, and nobody had any answers for me," she
says. "Doctors couldn't help me. I was spending all this cash and was
going broke, and when I got my last test results back and all my counts
were just horrible, I knew right then and there that this was the
end."
"I had outlived so many other people already," she says, having lost
friends from Lyme support groups, including some who just couldn't take
the suffering any more. "I didn't care if I was going to see my next
birthday. It's just enough. I was ready to call it a life and be done
with it."
So she packed up everything and moved to California to die. And she almost did.
Less than a week after moving, Ellie was attacked by a swarm of Africanised bees.
Ellie was in California for three days before her attack. "I wanted to
get some fresh air and feel the sun on my face and hear the birds
sing. I knew that I was going to die in the next three months or four
months. Just laying there in bed all crumpled up… It was kind of
depressing."
At this point, Ellie was struggling to stand on her own. She had a
caregiver on hand to help her shuffle along the rural roads by her place
in Wildomar, the place where she had chosen to die.
She was just standing near a broken wall and a tree when the first bee
appeared, she remembers, "just hitting me in the head". "All of a
sudden – boom! – bees everywhere."
Her caregiver ran. But Ellie couldn't run – she couldn't even walk.
"They were in my hair, in my head, all I heard was this crazy buzzing in
my ears. I thought: wow, this is it. I'm just going to die right
here."
Ellie, like 1–7 per cent of the world's population, is severely
allergic to bees. When she was two, a sting put her into anaphylaxis, a
severe reaction of the body's immune system that can include swelling,
nausea and narrowing of the airways. She nearly died. She stopped
breathing and had to be revived by defibrillation. Her mother drilled a
fear of bees into her to ensure she never ended up in the same dire
situation again. So when the bees descended, Ellie was sure that this
was the end, a few months earlier than expected.
Bees – and some other species in the order Hymenoptera, such as ants
and wasps – are armed with a potent sting that many of us are all too
aware of. This is their venom, and it's a mixture of many compounds.
Perhaps the most important is a tiny 26-amino-acid peptide called
melittin, which constitutes more than half of the venom of honey bees
and is found in a number of other bees and wasps. This little compound
is responsible for the burning pain associated with bee stings. It
tricks our bodies into thinking that they are quite literally on fire.
When we experience high temperatures, our cells release inflammatory
compounds that activate a special kind of channel, TRPV1, in sensory
neurons. This ultimately causes the neurons to send a signal to the
brain that we're burning. Melittin subversively makes TRPV1 channels
open by activating other enzymes that act just like those inflammatory
compounds.
Jellyfish and other creatures also possess TRPV1-activating compounds
in their venoms. The endpoint is the same: intense, burning pain.
"I could feel the first five or ten or fifteen but after that... All
you hear is this overwhelming buzzing, and you feel them hitting your
head, hitting your face, hitting your neck," says Ellie.
"I just went limp. I put my hands up and covered my face because I
didn't want them stinging me in the eyes… The next thing I know, the
bees are gone."
When the bees finally dissipated, her caregiver tried to take her to
the hospital, but Ellie refused to go. "This is God's way of putting me
out of my misery even sooner," she told him. "I'm just going to accept
this."
"I locked myself in my room and told him to come collect the body tomorrow."
But Ellie didn't die. Not that day, and not three to four months later.
"I just can't believe that was three years ago, and I just can't
believe where I am now," she tells me. "I had all my blood work done.
Everything. We tested everything. I'm so healthy."
She believes the bees, and their venom, saved her life.
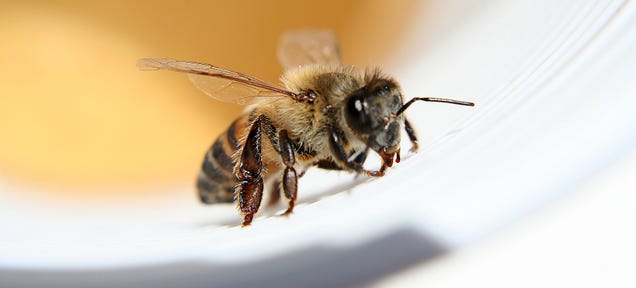
The idea that the same venom toxins that cause harm may also be used
to heal is not new. Bee venom has been used as a treatment in East Asia
since at least the second century BCE. In Chinese traditional medicine,
scorpion venom is recognised as a powerful medicine, used to treat
everything from eczema to epilepsy. Mithradates VI of Pontus, a
formidable enemy of Rome (and also an infamous toxinologist), was said
to have been saved from a potentially fatal wound on the battlefield by
using steppe viper venom to stop the bleeding.
"Over millions of years, these little chemical engineers have
developed a diversity of molecules that target different parts of our
nervous system," says Ken Winkel, Director of the Australian Venom
Research Unit at the University of Melbourne. "This idea of applying
these potent nerve toxins to somehow interrupt a nervous disease has
been there for a long time. But we haven't known enough to safely and
effectively do that."
Despite the wealth of history, the practical application of venoms in
modern therapeutics has been minimal. That is, until the past ten years
or so, according to Glenn King at the University of Queensland in
Brisbane, Australia. In 1997, when Ellie was bouncing around from doctor
to doctor, King was teasing apart the components of the venom from the
Australian funnel-web, a deadly spider. He's now at the forefront of
venom drug discovery.
King's group was the first to put funnel-web venom through a
separation method called high-performance liquid chromatography (HPLC),
which can separate out different components in a mixture based on
properties like size or charge. "I was just blown away," he says. "This
is an absolute pharmacological goldmine that nobody's really looked at.
Clearly hundreds and hundreds of different peptides."
Over the course of the 20th century, suggested venom treatments for a
range of diseases have appeared in scientific and medical literature.
Venoms have been shown to fight cancer, kill bacteria, and even serve as
potent painkillers – though many have only gone as far as animal
tests. At the time of writing, just six had been approved by the US
Food and Drug Administration for medical use (one other – Baltrodibin,
adapted from the venom of the Lancehead snake – is not FDA approved,
but is available outside the US for treatment of bleeding during
operations).
The more we learn about the venoms that cause such awful damage, the
more we realise, medically speaking, how useful they can be. Like the
melittin in bee venom.
Melittin does not only cause pain. In the right doses, it punches
holes in cells' protective membranes, causing the cells to explode. At
low doses, melittin associates with the membranes, activating
lipid-cutting enzymes that mimic the inflammation caused by heat. But at
higher concentrations, and under the right conditions, melittin
molecules group together into rings creating large pores in membranes,
weakening a cell's protective barrier and causing the entire cell to
swell and pop like a balloon.
Because of this, melittin is a potent antimicrobial, fighting off a
variety of bacteria and fungi with ease. And scientists are hoping to
capitalise on this action to fight diseases like HIV, cancer, arthritis
and multiple sclerosis.
For example, researchers at the Washington University School of
Medicine in St Louis, Missouri, have found that melittin can tear open
HIV's protective cell membrane without harming human cells. This
envelope-busting method also stops the virus from having a chance to
evolve resistance. "We are attacking an inherent physical property of
HIV," Joshua L Hood, the lead author of the study, said in a press
statement. "Theoretically, there isn't any way for the virus to adapt to
that. The virus has to have a protective coat." Initially envisioned
as a prophylactic vaginal gel, the hope is that melittin-loaded
nanoparticles could someday be injected into the bloodstream, clearing
the infection.
Ellie is the first to admit that her tale sounds a little tall. "If
someone were to have come to me and say, 'Hey, I'll sting you with some
bees, and you'll get better', I would have said, 'Absolutely not!
You're crazy in your head!'" But she has no doubts now.
After the attack, Ellie watched the clock, waiting for anaphylaxis to
set in, but it didn't. Instead, three hours later, her body was racked
with pains. A scientist by education before Lyme took its toll, Ellie
thinks that these weren't a part of an allergic response, but instead
indicated a Jarisch–Herxheimer reaction – her body was being flooded
with toxins from dying bacteria. The same kind of thing can happen when a
person is cured from a bad case of syphilis. A theory is that certain
bacterial species go down swinging, releasing nasty compounds that
cause fever, rash and other symptoms.
For three days, she was in pain. Then, she wasn't.
"I had been living in this… I call it a brown-out because it's like
you're walking around in a half-coma all the time with the inflammation
of your brain from the Lyme. My brain just came right out of that fog. I
thought: I can actually think clearly for the first time in years."
With a now-clear head, Ellie started wondering what had happened. So
she did what anyone else would do: Google it. Disappointingly, her
searches turned up very little. But she did find one small 1997 study by
scientists at the Rocky Mountain Laboratories in Montana, who'd found
that melittin killed Borrelia. Exposing cell cultures to purified melittin, they reported that the compound completely inhibited Borrelia
growth. When they looked more closely, they saw that shortly after
melittin was added, the bacteria were effectively paralysed, unable to
move as their outer membranes were under attack. Soon after, those
membranes began to fall apart, killing the bacteria.
Convinced by her experience and the limited research she found, Ellie
decided to try apitherapy, the therapeutic use of materials derived
from bees.
Her bees live in a "bee condo" in her apartment. She doesn't raise
them herself; instead, she mail orders, receiving a package once a week.
To perform the apitherapy, she uses tweezers to grab a bee and press
it gently where she wants to be stung. "Sometimes I have to tap them on
the tush a little bit," she says, "but they're usually pretty willing
to sting you."
She started on a regimen of ten stings a day, three days a week:
Monday, Wednesday, Friday. Three years and several thousand stings
later, Ellie seems to have recovered miraculously. Slowly, she has
reduced the number of stings and their frequency – just three stings in
the past eight months, she tells me (and one of those she tried in
response to swelling from a broken bone, rather than Lyme-related
symptoms). She keeps the bees around just in case, but for the past year
before I talked to her, she'd mostly done just fine without them.
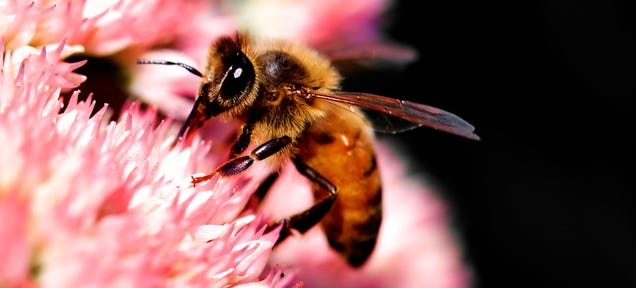
Modern science has slowly begun to take apart venoms piece by piece to
understand how they do the things they do, both terrible and
tremendous. We now know that most venoms are complex cocktails of
compounds, with dozens to hundreds of different proteins, peptides and
other molecules to be found in every one. The cocktails vary between
species and can even vary within them, by age, location or diet. Each
compound has a different task that allows the venom to work with
maximum efficiency – many parts moving together to immobilise, induce
pain, or do whatever it is that the animal needs its venom for.
The fact that venoms are mixtures of specifically targeted toxins
rather than single toxins is exactly what makes them such rich sources
of potential drugs – that's all a drug is, really, a compound that has a
desired effect on our bodies. The more specific the drug's action, the
better, as that means fewer side-effects.
"It was in the 2000s that people started saying well, actually,
[venoms] are really complex molecular libraries, and we should start
screening them against specific therapeutic targets as a source of
drugs," says King.
Of the seven venom-derived pharmaceuticals on the international
market, the most successful, captopril, was derived from a peptide found
in the venom of the Brazilian viper (Bothrops jararaca). This
venom has been known for centuries for its potent blood-thinning
ability – one tribe are said to have coated their arrow tips in it to
inflict maximum damage – and the drug has made its parent company more
than a billion dollars and become a common treatment for hypertension.
Bryan Fry, a colleague of Glenn King's at the University of Queensland
and one of the world's most prolific venom researchers, says the
captopril family and its derivatives still command a market worth
billions of dollars a year. Not bad for something developed in 1970s.
"It's not only been one of the top twenty drugs of all time," he says,
"it's been one of the most persistent outside of maybe aspirin."
And it's not just captopril. Fry points to exenatide, a molecule found
in the venom of a lizard, the gila monster, and the newest
venom-derived pharmaceutical on the US market. Known by the brand name
Byetta, this has the potential to treat type 2 diabetes, stimulating the
body to release insulin and slow the overproduction of sugar, helping
reverse the hormonal changes caused by the disease.
Rare cases like Ellie's are a reminder of the potent potential of
venoms. But turning folk knowledge into pharmaceuticals can be a long
and arduous process. "It could take as long as ten years from the time
you find it and patent it," says King. "And for every one that you get
through, ten fail."
Since the 1997 study, no one had looked further into bee venom as a potential cure for Lyme disease, until Ellie.
Ellie now runs a business selling bee-derived beauty products called
BeeVinity, inspired after, she says, noticing how good her skin looked
as she underwent apitherapy. "I thought, 'Well, people aren't going to
want to get stung with bees just to look good.'"
Ellie has partnered with a bee farm that uses a special electrified
glass plate to extract venom. As the bees walk across the plate on the
way to and from their hive, harmless currents stimulate the bees to
release venom from their abdomens, leaving teeny little droplets on the
glass, which are later collected. Ellie says it takes 10,000 bees
crossing that plate to get 1 gram of venom (other sources, such as the
Food and Agriculture Organization of the UN, quote 1 million stings per
gram of venom), but "those bees are not harmed".
For her, it is more than just a way to make a living: it's "an amazing
blessing". Proceeds from her creams and other products support bee
preservation initiatives, as well as Lyme disease research. In
addition, she sends some of the venom she purchases – which, due to the
cost of the no-harm extraction method she uses, she says is "more
expensive than gold" – to Eva Sapi, Associate Professor of Biology and
Environmental Science at the University of New Haven, who studies Lyme
disease.
Sapi's research into the venom's effects on Lyme bacteria is ongoing
and as yet unpublished, though she told me the results from preliminary
work done by one of her students look "very promising". Borrelia
bacteria can shift between different forms in the body, which is part
of what makes them so hard to kill. Sapi has found that other
antibiotics don't actually kill the bacteria but just push them into
another form that is more dormant. As soon as you stop the antibiotics,
the Borrelia bounce back. Her lab is testing different bee
venoms on all forms of the bacteria, and so far, the melittin venom
seems effective.
The next step is to test whether melittin alone is responsible, or whether there are other important venom components. "We also want to see, using high-resolution images, what exactly happens when bee venom hits Borrelia," Sapi told me.
She stresses that much more data is needed before any clinical use can
be considered. "Before jumping into the human studies, I would like to
see some animal studies," she says. "It's still a venom." And they
still don't really know why the venom works for Ellie, not least because
the exact cause of post-treatment Lyme disease symptoms remains
unknown. "Is it effective for her because it's killing Borrelia, or is it effective because it stimulates the immune system?" asks Sapi. It's still a mystery.
There's a long way to go for bee venom and melittin. And it takes a
lot of work – and money – to turn a discovery into a safe, working
medicine. But labs like King's are starting to tap the pharmaceutical
potential that lies in the full diversity of venomous species. And King,
for one, believes that scientists are entering a new era of drug
discovery.
In the past, venoms have been investigated because of their known
effects on humans. Such investigations required both knowledge of the
venom's clinical effects and large volumes of venom, so until now only
large species, like snakes, with easily extracted venoms have been
studied in any depth. But that's changing. Technological advances allow
for more efficient venom extraction as well as new ways to study
smaller amounts of venom. The preliminary tests for pharmaceuticals can
now start with nothing more than a genetic sequence. "We can now
genomically look at the toxins in these animals without having to
actually even purify the venom," says King, "and that changes
everything." Ken Winkel thinks venomous animals will be excellent drug
resources for devastating neurological diseases, as so many of their
venoms target our nervous system. "We really don't have great drugs in
this area," he says, "and we have these little factories that have a
plethora of compounds…"
No one knows exactly how many venomous species there are on this
planet. There are venomous jellyfish, venomous snails, venomous insects,
even venomous primates. With that, however, comes a race against time
of our own making. Species are going extinct every year, and up to a
third may go extinct from climate change alone.
"When people ask me what's the best way to convince people to preserve
nature, your weakest argument is to talk about how beautiful and
wonderful it is," says Bryan Fry. Instead, he says, we need to
emphasise the untapped potential that these species represent. "It's a
resource, it's money. So conservation through commercialisation is
really the only sane approach."
Ellie couldn't agree more. "We need to do a lot more research on these
venoms," she tells me emphatically, "and really take a look at what's
in nature that's going to help us."
This article first appeared on Mosaic and is republished here under Creative Commons license. Images by Danny Perez Photography, Vitor Sá, Matthew T Rader under Creative Commons license.